Exploring a Novel Fuel: My Ph.D. Journey in Combustion Science
Institution: McGill University
I am currently pursuing a Ph.D. in the Department of Mechanical Engineering at McGill University. Many times, when I explain my research, people respond with, “That doesn’t sound like mechanical engineering.” And they’re right. While my program is in mechanical engineering, and I am a fully licensed engineer in Canada practicing in the mechanical engineering discipline, I do not define myself solely by that title. I believe that solving real-world challenges requires expertise beyond a single discipline.
I also envision myself as a scientist rather than restricting myself to the label of a “mechanical engineer” confined to purely mechanical work. I embrace an interdisciplinary approach to drive innovation and strongly value collaboration, which is a key aspect of my current research. Multidisciplinarity is essential for addressing complex global challenges, and I strive to integrate knowledge from various fields to develop innovative solutions.
My Ph.D. research focuses on combustion, specifically exploring the science and physics of novel metal-fuel combustion. I am fortunate to work with my advisor Prof. Jeffrey Bergthorson and my “Science Father” Dr. Sam Goroshin — both of whom are the world leaders in metal-fuel combustion.
Metal as a fuel? If this concept sounds new (or even unbelievable), I invite you to read more!
What are the challenges of using renewable energy sources?
We all know that the energy crisis and reducing carbon emissions are among the most pressing challenges today. But why is it so difficult to transition to renewable energy sources and achieve large-scale decarbonization? This is a question I often receive from those outside the engineering field.
The challenge is not that we lack renewable energy sources — there is abundant solar energy in deserts and plentiful wind energy offshore. Instead, there are two major obstacles:
- Intermittency — Renewable energy is not always available. For instance, solar power is more abundant in the summer and significantly lower in the winter. However, in the 21st century, people expect continuous and reliable access to energy throughout the year.
- Geographical Distribution — Renewable energy sources are unevenly distributed across the globe as demonstrated by the map below from the Global Wind/Solar Atlas. What if people living in regions with low renewable energy potential (blue regions on the map) also need access to renewable power?
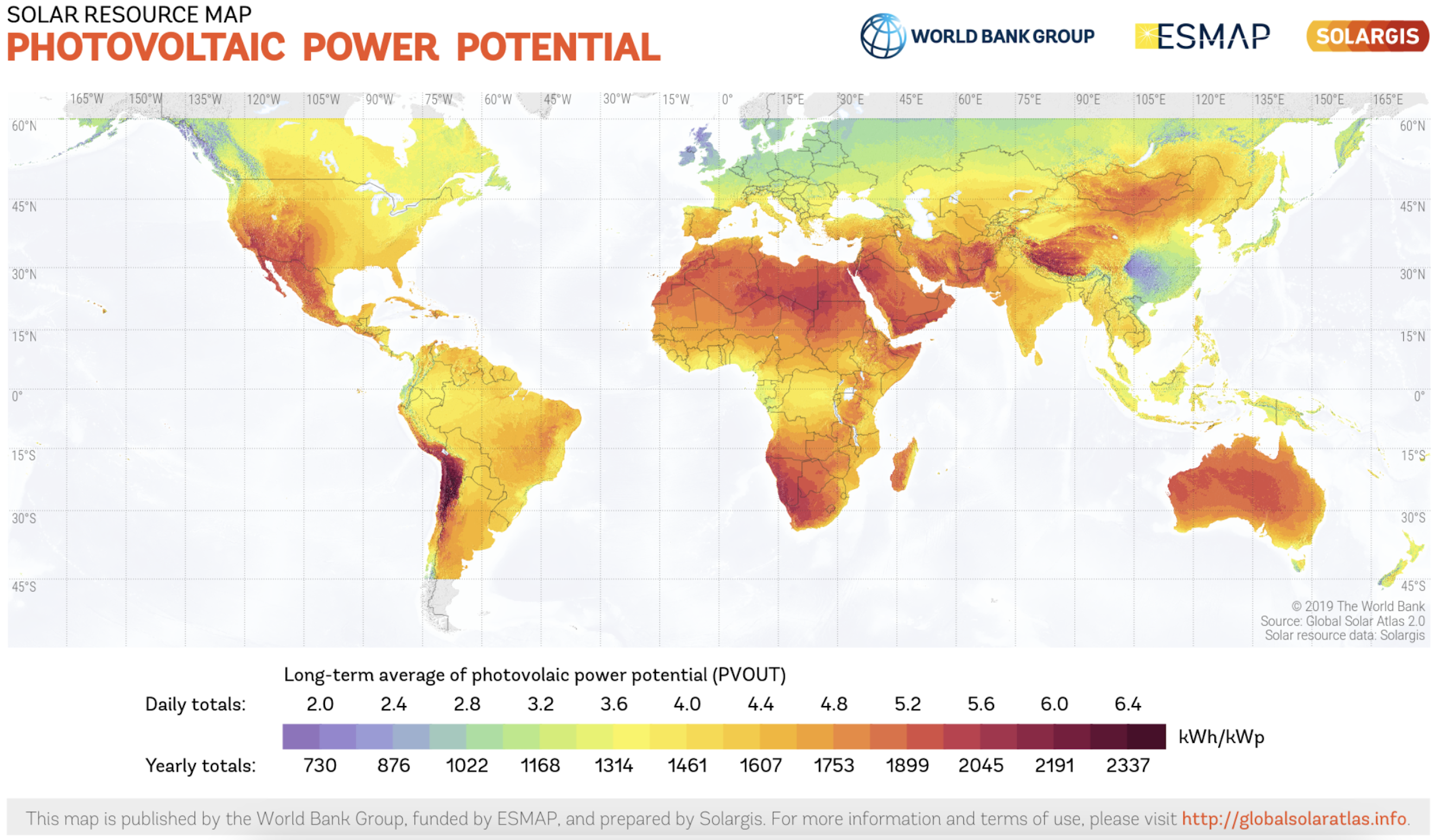
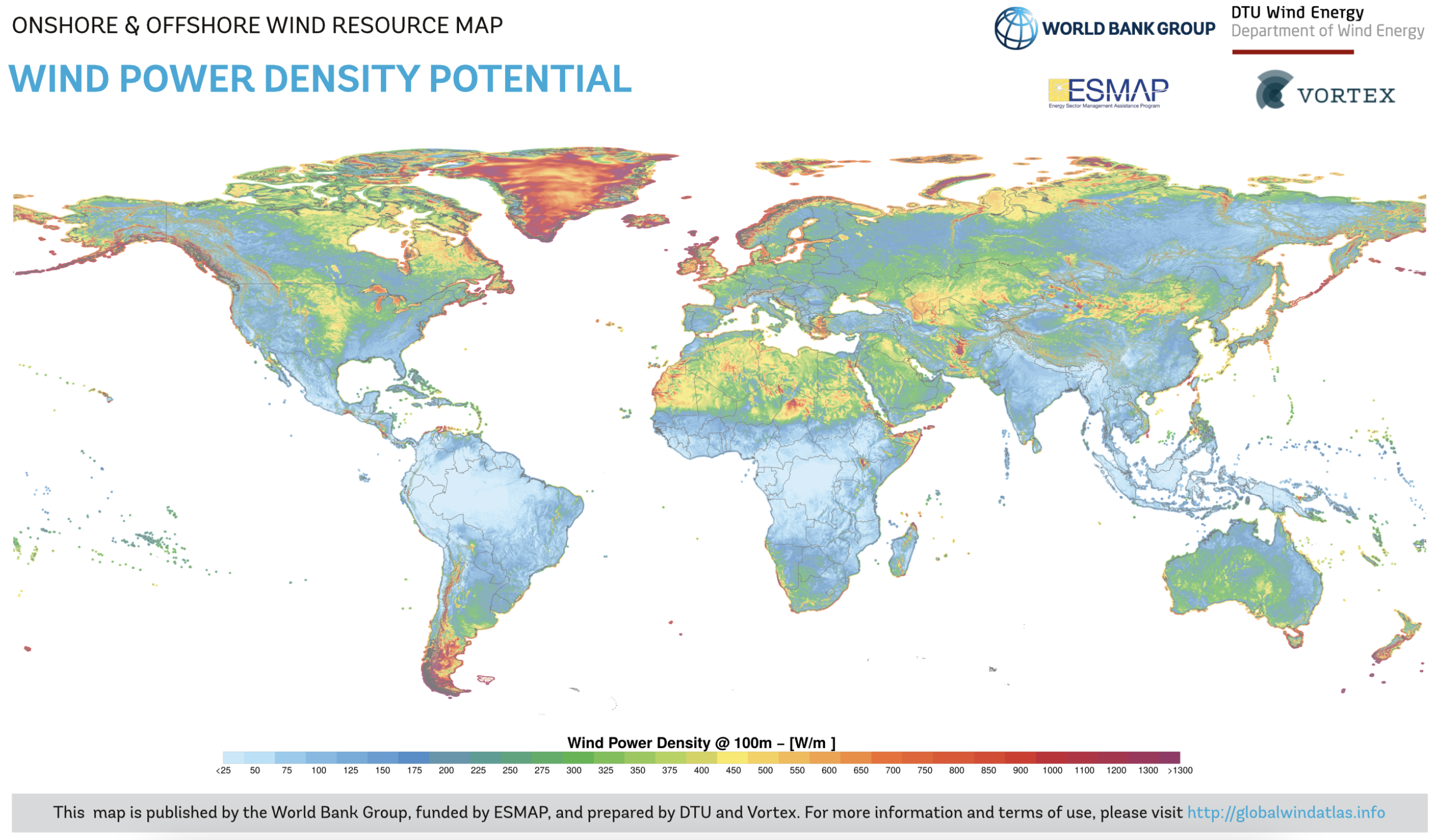
We need energy storage!
Since renewable energy sources are scattered geographically, what if we could "move" renewable energy around the globe? We cannot physically move wind or solar power, but we can convert them into electricity and transport that electricity. That sounds great, but it’s still not practical — we would need to build extremely long (and high-cost) electrical transmission lines to connect regions with abundant renewable energy sources to those with limited access.
What about we could store renewable energy instead? For example, We could store solar energy when solar intensity is high in the summer and then transport that stored energy to various locations for later use. Electrical energy itself cannot be stored. However, we can convert that electrical energy (converted from renewable sources) into chemical energy — a form of energy that can be transported and used when needed. A simple example is a battery — it stores energy chemically and can be used on demand. But why haven't batteries solved the energy crisis? Because batteries have a very low energy density — to perform high-energy-demand tasks like space heating, we would need an extremely large and heavy battery, which is impractical.
Another solution is hydrogen — it stores chemical energy like batteries and can be produced by splitting water molecules using renewable energy sources. Hydrogen combustion is carbon-free, and water is an abundant resource on Earth. However, the main challenge with hydrogen is its low volumetric energy density — meaning it also requires a very large storage space. This makes it difficult (and unsafe) to transport hydrogen efficiently from one location to another.
My Ph.D. research proposes a relatively new solution — metal fuels. Just as renewable energy (e.g. solar, wind) can be used to split water (H2O) into hydrogen (H2) and oxygen, we can also use renewable energy to reduce metal oxides (e.g. Al2O3, Fe2O3) into metal (Al, Fe) and oxygen! In other words, we are storing solar and wind energy inside the metal in the form of chemical energy. Metals can be transported easily, allowing us to move stored renewable energy around the globe without the need for long transmission lines. This transported metals can then be burned later when needed. That’s why we call metals energy carriers!
Think of metal fuels as a "better version of a battery" — a compact, high-energy-density energy carrier. The illustration below shows how aluminum, iron and silicon metals compare to batteries and hydrogen in terms of storage space required (based on their volumetric energy density), demonstrating how much smaller the storage space for metals can be for the same energy output.
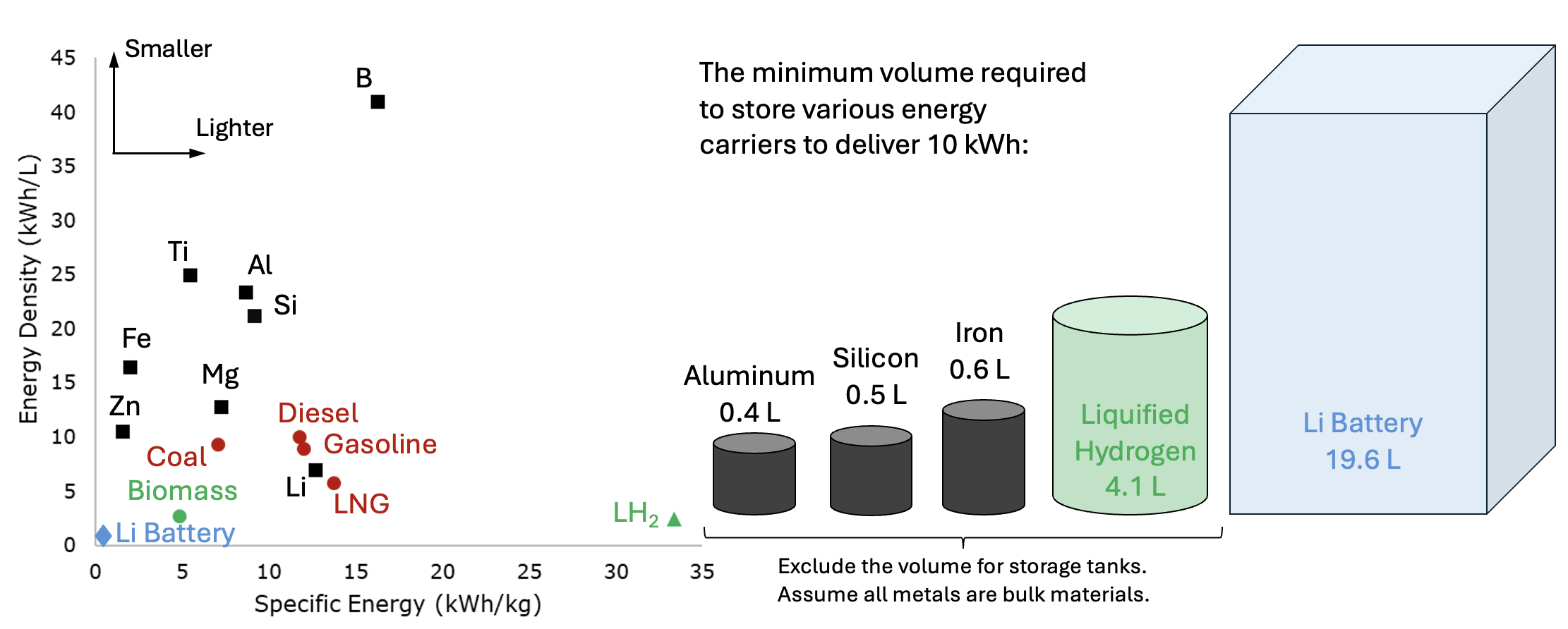
Metal fuels as energy carriers
What makes metal fuels particularly interesting is that they are carbon-free. When metal (e.g. Al) is burned, it produces metal oxides (e.g. Al2O3) without emitting greenhouse gases. Since the combustion products are solid metal oxides, they can be captured and recycled. These metal oxides can then be reduced back to pure metal, allowing them to be reused repeatedly in a closed sustainable loop, as shown below.
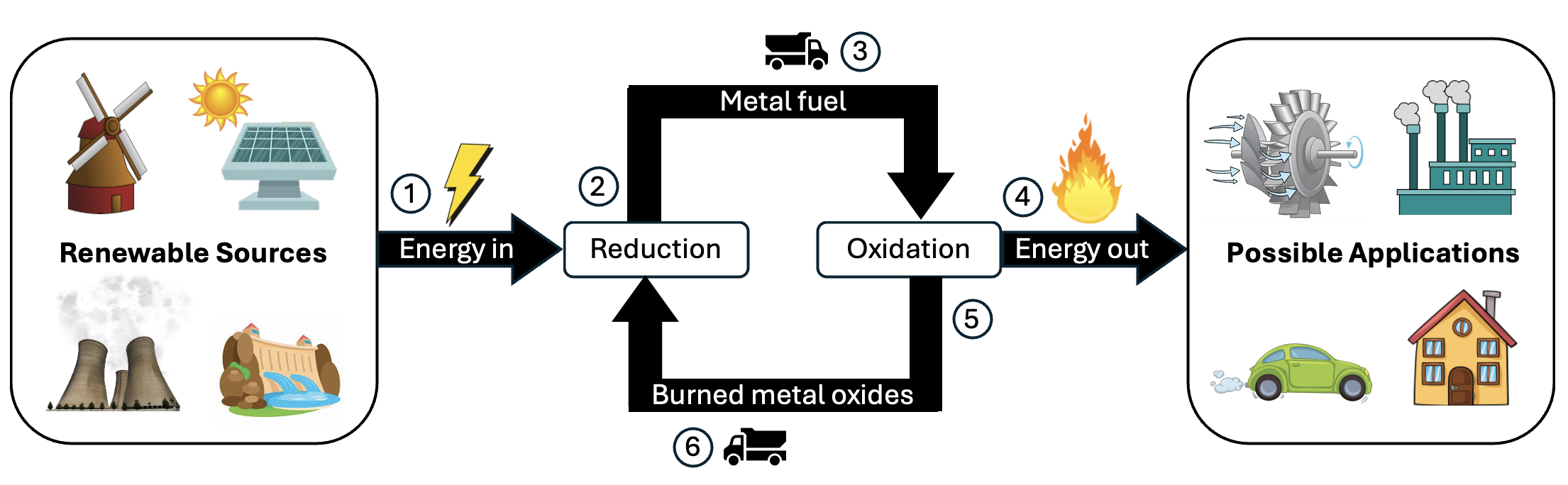
As of now, most research on metal fuels has focused on aluminum and iron. However, I have taken on the challenge of investigating a new metal fuel — silicon combustion (more like a metalloid). What makes silicon particularly exciting is that it is the second most abundant element on Earth (after oxygen). It is a promising energy carrier, but its combustion physics and chemistry remain largely unexplored. During my Ph.D. program, I developed a research framework that successfully stabilized silicon flames, enabling a detailed study of their combustion behaviours. Stay tuned — some publications on this topic are coming soon! :)
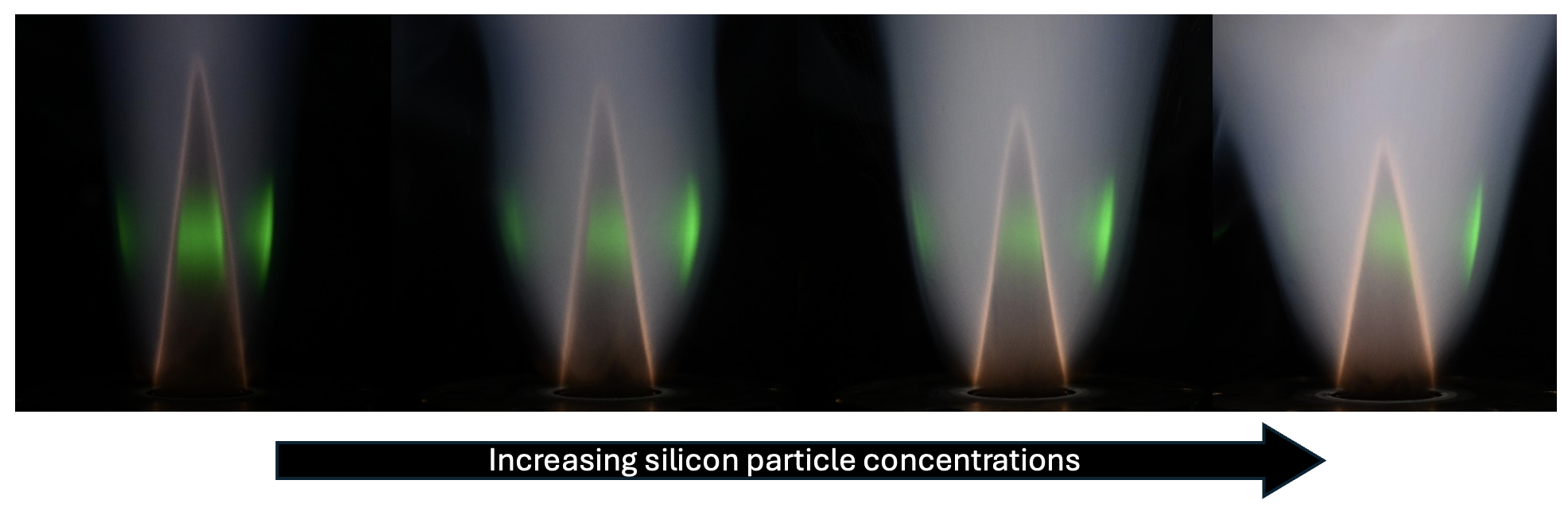
Common myths and FAQs about metal fuels
Metal fuels are a fascinating innovation, but as a relatively new concept, misconceptions about their role as energy carriers are common. Based on my experiences at various international conferences and interactions with people across various disciplines, here are some frequently asked questions about metal fuels.
Iron rusting is also an oxidation process that releases heat, but the heat released is often unnoticeable because it is a slow oxidation process.
Electricity cannot be stored directly. Hydrogen stores energy chemically, but requires large storage space and poses safety risks such as leakage. Metal fuels can be seen as a 'power bank' — they store energy efficiently and can be easily transported and used as needed.
For silicon fuel, which I am currently researching, sustainability may not be a concern for long term as it is abundant and can be extracted from sand.
Compared to existing solutions, the main challenge of metal fuels is their technology readiness, as they remain a relatively new and emerging concept. Therefore, advancing this field requires more passionate and innovative researchers to push the technology forward :)